Hamstring injuries in football, an unsolved puzzle
VOETBAL MEDISCH SYMPOSIUM 2020
DE BEHANDELING VAN VOETBALBLESSURES
PRAKTISCHE WETENSCHAP
OP DE KNVB CAMPUS IN ZEIST VINDT KOMEND JAAR OPNIEUW HET VOETBALMEDISCH SYMPOSIUM PLAATS.
HET SYMPOSIUM IS DÉ PLEK OM COLLEGA’S BINNEN HET VOETBALMEDISCHE DOMEIN TE ONTMOETEN OF KENNIS OP TE DOEN VAN GERENOMMEERDE EXPERTS. EN DIE NIEUWSTE INNOVATIES TE ZIEN OP HET GEBIED VAN VOETBALMEDISCHE EN FYSIEKE PRESTATIES.
NA VORIG JAAR DE DIAGNOSTIEK VAN VOETBALBLESSURES BELICHT TE HEBBEN, ROLT DE BAL DIT JAAR VERDER NAAR DE BEHANDELING VAN VOETBALBLESSURES. HET INHOUDELIJKE PROGRAMMA BIEDT OPNIEUW SPREKERS DIE ZICH ONDERSCHEIDEN IN ZOWEL DE DAGELIJKSE ZORG VOOR DE VOETBALLERS ALS OP WETENSCHAPPELIJK GEBIED.
VOETBAL MEDISCHE WORKSHOP 2020
(VELD)REVALIDATIE NA EEN VOETBALBLESSURE
OP 4 MAART ZAL ER WEDEROM EEN WORKSHOP PLAATS VINDEN BIJ HET KNVB VOETBAL MEDISCH CENTRUM.
OOK DIT JAAR BELOOFD HET EEN OCHTENDVULLEND PROGRAMMA TE ZIJN WAAR VOORNAMELIJK (SPORT)FYSIOTHERAPEUTEN HUN KENNIS MEE KUNNEN UITBREIDEN.
TIJDENS DE WORKSHOP ZAL MATT TABERNER ZIJN KENNIS EN EXPERTICE MET DE DEELNEMERS GAAN DELEN. MATT TABERNER IS EEN ERVAREN CLINICUS DIE AL JAREN EINDVERANTWOORDELIJK IS VOOR DE REVALIDATIE VAN TOPVOETBALLERS IN DE PREMIER LEAGUE. ZIJN FOCUS LIGT VOORNAMELIJK OP FYSIEKE ONTWIKKELING EN PRESTATIES. TEVENS IS HIJ DE ONTWIKKELAAR VAN HET ‘CONTROL-CHAOS CONTINUUM’.
DIT FRAMEWORK, WELKE VIJF FASES BESCHRIJFT HOE DE VELDREVALIDATIE NA EEN VOETBALBLESSURE OPGEBOUWD KAN WORDEN, STAAT CENTRAAL BINNEN DE WORKSHOP. DE THEORETISCHE ACHTERGROND,
DE TOEPASSING EN HET PRAKTISCHE ASPECT ZULLEN ALLEN AAN BOD KOMEN TIJDENS DE WORKSHOP.
reviews
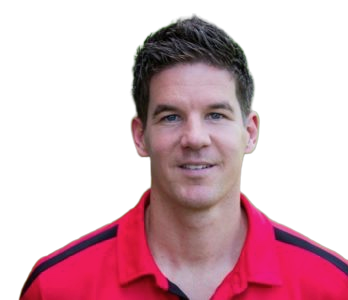
Nick van der Horst
Meet the soccerdoc
Nick van der Horst behaalde zijn diploma fysiotherapie in 2007 aan de Hogeschool Utrecht. Hij werkte 10 jaar lang als sportfysiotherapeut/echografist/docent bij het Academie Instituut te Utrecht. Daarna heeft hij de overstap gemaakt naar waar zijn hart ligt, het professionele voetbal. Hij heeft twee jaar als sportfysiotherapeut en hoofd van de medische staf bij Go Ahead Eagles in Deventer gewerkt. Momenteel is is Nick werkzaam bij de KNVB. Zijn onderzoeks-activiteiten zijn gefocust op de voetbal-medische zorg. In 2017 behaalde hij zijn doctoraal na het verdedigen van zijn proefschrift ‘Prevention of hamstring injuries in male soccer’.
Part 1: Anatomy, biomechanics and injury risk factors
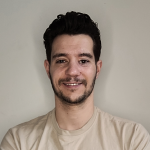
Blogger: Raúl Gómez
As example of what I just said, the research carried out by Ekstrand et al. (2016, 2022) showed an increase in hamstring injuries from the 2001/02 season to the 2021/22 (Image 1A and 1B). However, in the following image (Image 2) we can see how the number of published scientific articles has increased considerably too. In this case, I have written “Hamstring injury” in Pubmed (580 published articles in 2021).
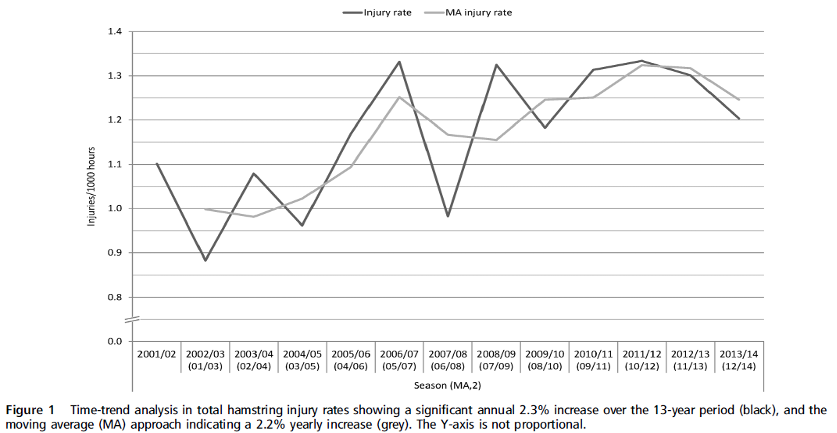
Image 1A. Increase in hamstring injuries from 2001/02 to 2013/14 season. (Ekstrand, et al., 2016)
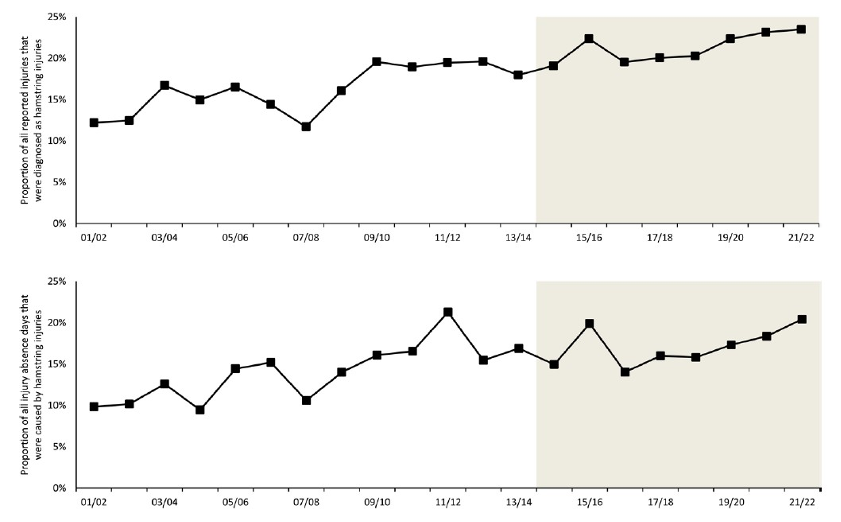
Image 1B. Proportion of all reported injuries that were diagnosed as hamstring injuries (top) and the proportion of all injury absence days caused by hamstring injuries (bottom) (Ekstrand et al., 2022)
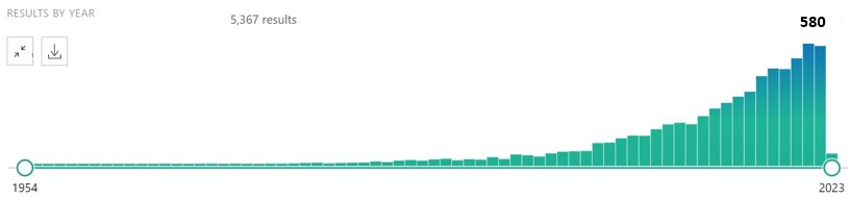
Image 2.
What makes this muscle group so vulnerable to injury? What changes occur in players who suffer hamstring muscle tears? Why is it the most recurrent injury in soccer? I will try to solve these questions and many others but, before I start, I want to make it clear that there are no universal answers, there is no cure-all exercise and no single method that works the same for all players and teams. As sports professionals we must know how to find the best way for each person with whom we work. I hope this blog is a useful guide for sport and physical therapy professionals, but we must never forget that an injury has a multifactorial origin and we must know how to work together with other professionals such as doctors, physiotherapists or nutritionists.
Hamstrings Anatomy
The hamstring muscle complex (Image 3) is comprised of 3 muscles, semimembranosus (SM), semitendinosus (ST) and biceps femoris (BF), the latter divided into long head (BFlh) and short head (BFsh). Although I will analyse everything in much more detail below, in table 1 you can see the origin, insertion and main functions of each muscle (Rodgers & Raja, 2020).
Origin
According to Stephen et al., (2019) all the hamstring muscles originate in the ischial tuberosity (IT). ST and BFlh share origin forming a conjoint tendon that originates in the posteromedial aspect of the IT, while the SM muscle originates in the anteromedial aspect.
The BFsh is the only one that does not have a proximal attachment on the IT. Its origin is located on the lateral lip of the linea aspera, on the medial third of the femur.
Insertion
Distal attachments of all hamstring muscles are located in the knee, but with greater variation in location than in the hip. BFlh attaches using a direct and an anterior arm and different fascial connections (James, et al., 2015). The direct arm attaches on the lateral aspect of the fibular head, lateral to the styloid process and the anterior arm does it lateral to the insertion of the fibular collateral ligament on the fibular head.
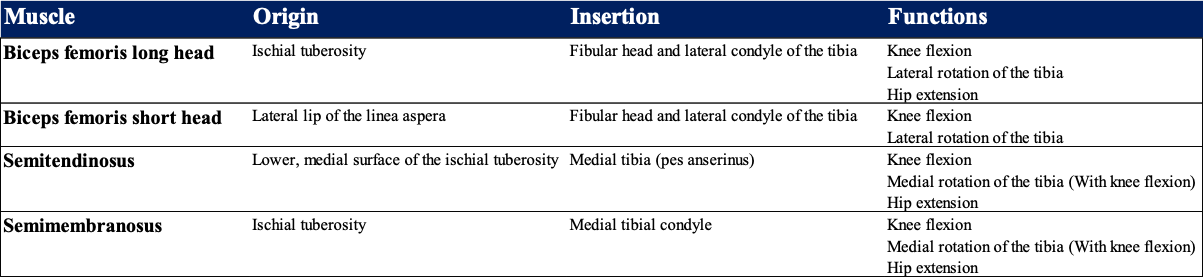
Table 1. Origin, insertion and functions of hamstrings muscles. (Rodgers & Raja, 2020)
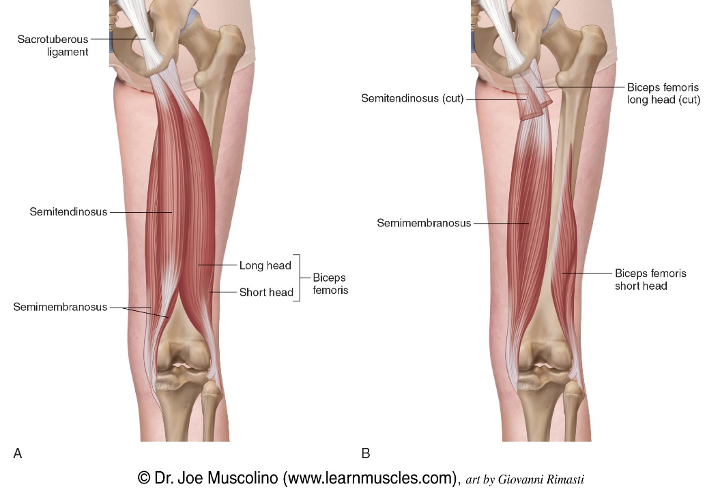
Image 3. Hamstring muscle complex
BFsh has several connections: the anteromedial side of the BFlh, posterolateral aspect of the joint capsule, capsulo-osseous layer of the iliotibial band, a lateral aponeurosis, and a direct and anterior arm which attach to the fibular head and lateral condyle of the tibia, respectively (James, et al., 2015).
The insertions of ST and SM are located on the medial side of the knee. The ST muscle joins with gracilis and sartorius forming a conjoint tendon called the pes anserinus (Image 4), which attaches to the anteromedial aspect of the proximal tibia (LaPrade, et al., 2015).
The posteromedial corner of the knee is a complex area in which the SM plays an important role, since it has several attachments and functions on the knee. Although in scientific research several connections of the distal SM tendon have been described, only in the next 3 (Image 5) there seems to be general agreement among researchers (De Maeseneer, et al., 2014): The direct arm of the SM, which attaches to the posterior aspect of the tibia, 1 cm below the joint. The anterior arm, which inserts deep to the medial collateral ligament and third, an expansion of the oblique popliteal ligament.
Apart from these connections, several more have been observed, up to 8 different expansions (Benninger & Delamarter, 2013), but not all researchers agree on them. Benninger & Delamarter (2013) observed that the oblique popliteal ligament does not really have a bony origin, but is indigenous to the semimembranosus tendon and therefore, they propose a change in terminology from ligament to tendon. This would also result in a significant clinical change due to the proprioceptive function of the tendon, which would mean a greater role of the SM in posterior knee stability.
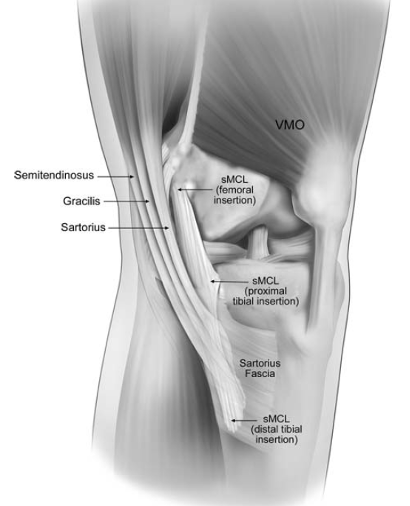
Image 4. ST, gracilis and sartorius common insertion (LaPrade, et al., 2015)
I can’t name all the connections from each article in this post, but I recommend taking a look at the bibliography for anyone interested in hamstring anatomy.
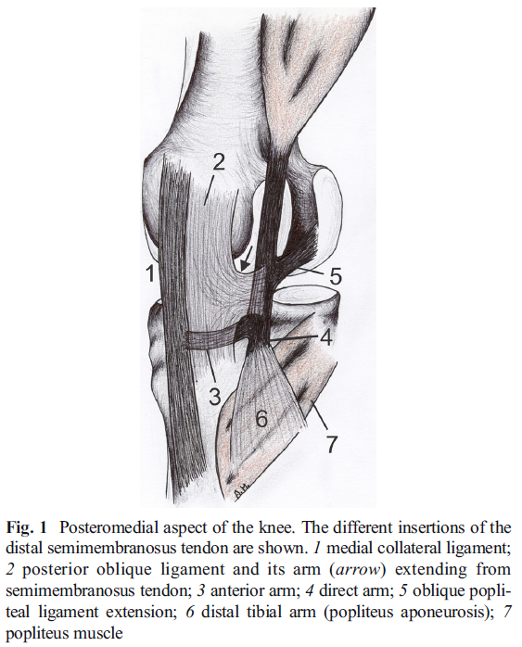
Image 5. Figure and description reproduced from De Maeseneer et al.(2014)
Functions
During the gait cycle, they coordinate hip extension and prevent excessive extension of the knee (Stepien, et al., 2019), specifically during the late swing phase, where they perform an eccentric contraction undergoing a period of power absorption (Schache, et al., 2012).
The hamstrings also have an important function on the muscular balance of the lower limb. Its coactivation during contraction of the quadriceps femoris muscle balances the lower limb and reduces the anterior shear forces on the tibiofemoral joint preventing posterior tibial translation (Stepien, et al., 2019) and, consequently, reducing the load on the anterior cruciate ligament (ACL) and protecting the knees with deficiencies in this ligament (Azmi, et al., 2018).
Due to its proximal origin in the IT and its connection with the sacrum via the sacrotuberous ligament, the hamstrings also perform a lumbo-pelvic stabilization function (Image 6) (Panayi, 2010), therefore, proper trunk and hip function will be a priority during the training process.
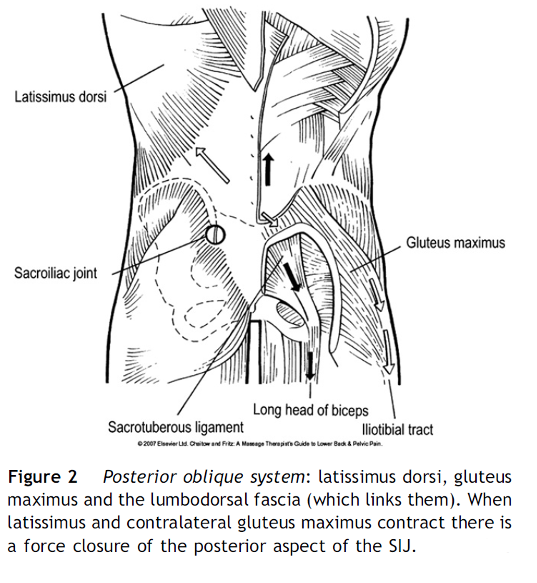
Image 6. Figures and descriptions reproduced from Panayi (2010)
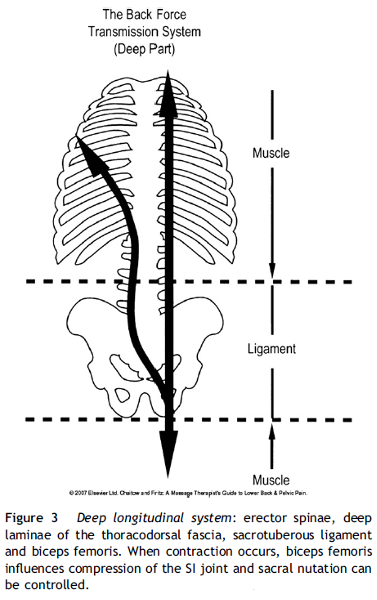
Proximally, the BF influences compression of the sacroiliac joint, helping to control sacral nutation (Panayi, 2010). Distally, its contraction externally rotates tibia and fibula because of its lateral attachment on the knee joint. The BF seems to be the muscle which contributes the most providing resistance to tibial internal rotation torque and anterior shear forces at the knee. Electrical stimulation of BF reduces considerably peak value of tibial internal rotation and anterior shear force, incrementing overall knee compressive force and, consequently, reducing anterior tibial translation and peak forces in the ACL, what in theory, might increase lateral stability in ACL-deficient knees (Azmi, et al., 2018).
In the medial side of the knee, ST and SM perform an antagonistic function to the BF in the transverse plane, they rotate the tibia internally and therefore, also provide resistance to tibial external rotation (Image 7). These two muscles play a crucial role in the posteromedial stability of the knee.
Toor, et al. (2019) carried out a study in which they dissected 8 cadaver knees to evaluate the role of the hamstring muscles in medial knee stability. To do this, they applied external forces in the 3 primary planes of movement (Sagittal, frontal and transverse) in various knee flexion positions (0º, 30º and 60º) with different load conditions in which they unloaded the force exerted by gracilis and semitendinosus individually and both in combination.
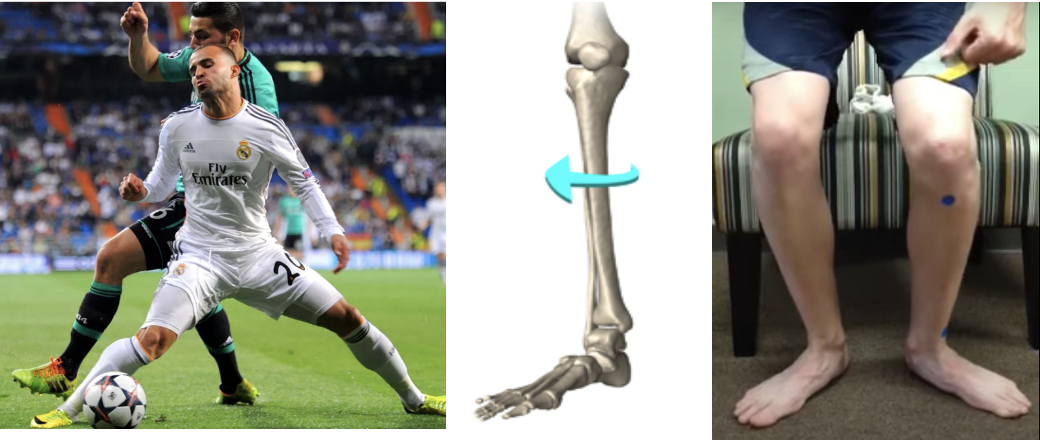
Image 7. Tibial external rotation
The results showed that unloading the force exerted by medial hamstrings increased external rotation and anterior tibial translation at all knee flexion angles together with an increase in valgus with the knee flexed 30º, what again, highlights the importance of the hamstrings to prevent ACL injuries.
Furthermore, an effect of the hamstrings on the meniscus has also been observed, specifically the SM retracts the medial meniscus posteriorly (De Maeseneer, et al., 2014). During knee flexion the meniscus moves backward to adapt to the shape and position of the femoral condyle. Thus, the load can be uniformly transferred, and damage to the meniscus can be prevented (Chen, et al., 2014).
Mechanism of hamstring strain
Due to their anatomy and biomechanics the hamstrings are a paramount muscle group in sports that require high intensity running actions and sprints. But, as we already know, they have a high risk of injury. But how do they get injured and why so many times?
There are 2 mechanisms of hamstring injury, stretch-type and sprint-type (Danielsson, et al., 2020). Stretch-type normally occurs during an excessive hip flexion with simultaneous knee extension (Image 8) and SM proximal tendon seems to be the most affected location. This type of injury is also associated with the strain of other muscles as quadratus femoris, adductor magnus, ST and BFlh and can finish with sports careers due to chronic pain and discomfort after injury (Askling & Saartok, 2008). This type of hamstring injury is common in dancers and sports which require large range of motion movements of the lower body.
Despite in soccer this type of injury is not very common, it can also occur during kicking, slide tackles or overhead controls.
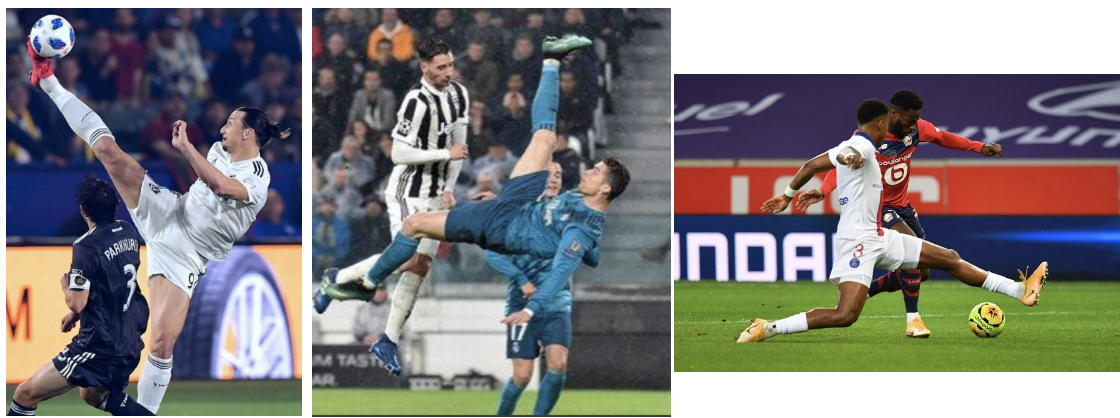
Image 8. Large hip flexion with knee extension in football actions
Injury type influences recovery time, being stretch type the one that requires longer periods. In addition, injuries located closer to the ischial tuberosity need longer recovery times too (Askling & Saartok, 2008).
Sprint-type injuries are the ones that occur the most, especially in sports such as soccer, where they can account for up to 80% of sustained hamstring injuries (Danielsson, et al., 2020). The most common location in this type of injury is the BFlh, especially in the proximal region. (Huygaerts, et al., 2020).
The hamstrings play a critical role in high intensity running. According to Morin et al. (2015), the hamstrings have a fundamental role in the production of horizontal force during the sprint. They observed that subjects with higher electromyographic activity during the final swing phase of sprint and a higher knee flexion eccentric peak torque showed a greater amount of horizontal ground reaction forces, which is related to better sprint performance.
Ishoi et al. (2019) also carried out a study on the influence of hamstring muscle peak torque and the ratio of torque development on sprint performance in soccer players. The results showed that hamstring torque production in the first 100 ms, but not from 100 ms to 200ms (Measured with dynamometer), was positively correlated to horizontal force production and maximum horizontal power production. In addition, early development of hamstring torque was related to better 30-m sprint times. But on the contrary, these authors found no association with maximum speed during sprinting.Therefore, the authors suggested that the rate of force development of the hamstrings is more important than their maximum force capacity in sprint performance.
Therefore, we can confirm the importance of this muscle group during sprinting, in which they participate by performing both hip extension and knee flexion torque to counteract the powerful movements of the hip and knee (Flexion and extension, respectively) in a very short period of time in which they can support 8 times the athlete’s body weight (Morin, et al., 2015).
This great role during sprint makes them very prone to injury due to the high eccentric load they support. Although there are some differences, most authors agree on the final phase of the swing as the moment of muscle injury, although other moments have also been observed, such as the early stance phase (Image 9).
In the final phase of the swing, the hip reaches its maximum flexion as the same time that the knee extends. After this, in preparation for foot strike, the hip starts to extend and the knee starts to flex (Kenneally-Dabrowski, et al., 2019). In this transition, the hamstrings endure great musculotendinous strain that coincides with the position of maximum elongation of the hamstrings during sprint due to simultaneous hip flexion and knee extension (Schache, et al., 2012). Therefore, hamstring muscles support high amount of negative work (Eccentric) in a short period of time, what makes them vulnerable to injury.
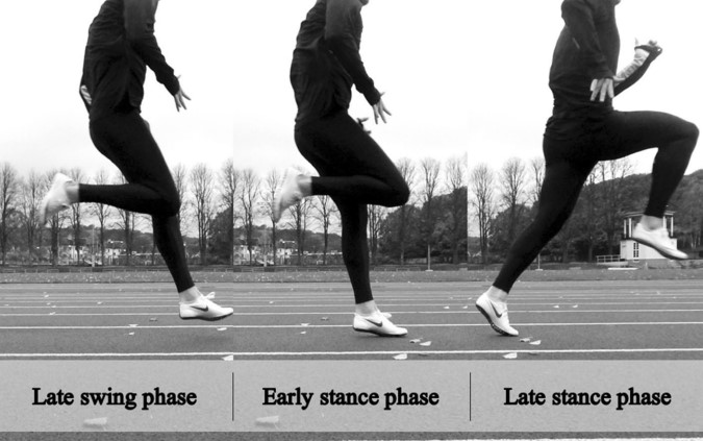
Image 9. The running gait cycle (Danielsson, et al., 2020)
Most researchers agree on this as the mechanism and moment of hamstring injury, but we can also find different conclusions if we continue our research.
In the review written by Kenneally-Dabrowski et al. (2019), hamstring injury mechanism during late swing and early stance was discussed. Regarding to early stance, some researchers have proposed that the greatest knee flexion and hip extension moments occur during this phase, when high opposing forces result from ground reaction forces as the foot strikes the floor (Orchard, 2012). Besides, Orchard (2012) argues that although muscle failure can occur in the final phase of the swing, this does not necessarily mean that the injury occurs at that time, but when that muscle, which has already failed, bears high loads in the early stance. This same author also suggests that muscle strains do not occur in open kinetic chain actions. He arguments that athletes performing upper limb open chain activities do not have a high muscle strain injury rate despite high-speed actions and this would also happen with the hamstrings, but there is no evidence to support this.
Other researchers propose a period instead of two phases, the swing-stance transition period (Liu, et al., 2017). These authors suggest that the hamstrings develop force on the hip and knee throughout the whole movement to counteract both the inertia of the lower leg during the late swing and ground reaction forces in early stance. Thus, it could be considered as a single period.
Although almost all research assumes that the hamstrings perform an eccentric action during high intensity running, a few years ago Van Hooren and Bosch (2017) challenged this claim with a rather interesting theory.
According to their theory, the contractile element of the muscle (Muscular fascicles) would not perform an eccentric but isometric action, while the series elastic element (Tendons, aponeurosis and fascial and connective tissue) would elongate and then recoil in preparation for ground contact. In this case, the injury would be caused by the muscular inability to maintain the isometric action when external forces are too high, so the muscle would elongate in an eccentric contraction that could lead to injury. Furthermore, a loss of coordination in the pelvic area is also proposed as an injury mechanism, since it can increase the distance between muscle attachments, also causing an eccentric muscle action.
To explain the muscular elongation of the hamstrings during the gait cycle, they introduce the concept of “muscle slack” (Image 10), which is defined as the delay between the contraction of the muscle fibers and the beginning of the stretching of the series elastic elements. (Van Hooren & Bosch, 2016). As an example, we could compare it to how an elastic band works when we pull it. When we start to pull, it offers minimal or no resistance until it stretches. Once it has enough tension, it begins to generate force and resistance. The same would happen in the hamstring muscles, which first would be in a relaxed position until they receive the neuromuscular signal that activates muscle contraction. This would take out the muscle slack to align the muscle tendon unit to the point where the force is transmitted to the elastic elements in series.
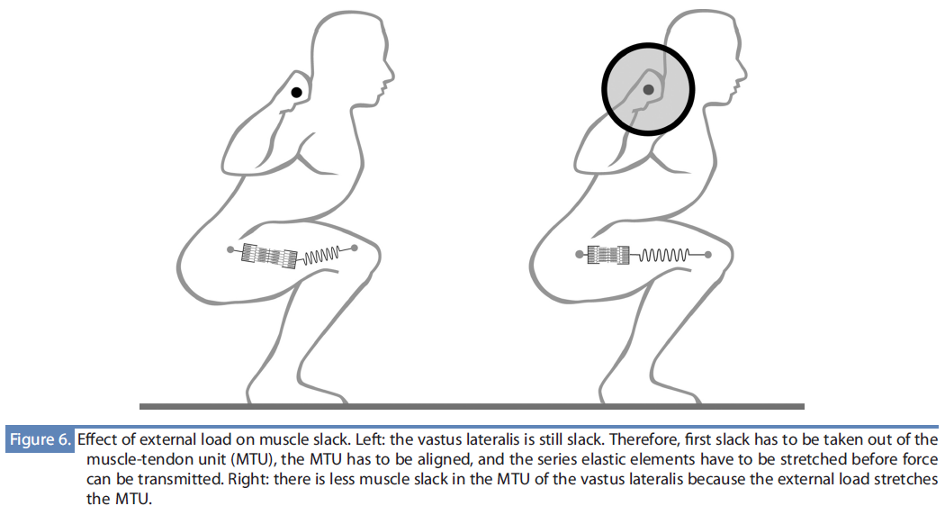
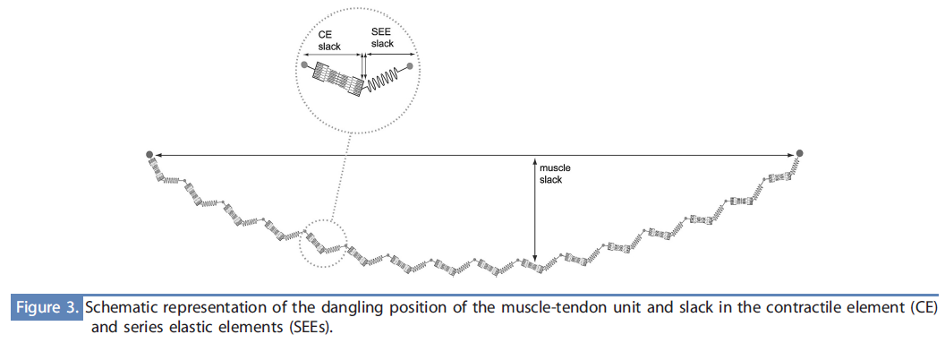
Image 10. Images and descriptions reproduced from Van Hooren & Bosch (2016)
Therefore, hamstring lengthening during high intensity running, according to this theory, would be the result of the alignment of the musculotendinous unit, taking out the muscle slack to place the muscle close to its optimal length to generate force in an isometric action.
More evidence is needed about the latter theory, which challenges the current knowledge about mechanism of injury and biomechanical assessment models.
However, there does seem to be a general agreement on the most common location in sprint-type injuries, BFlh is the muscle with the highest number of injuries in all investigations, let’s see why.
Both architecture and biomechanics vary between muscles of synergistic groups to have a greater capacity to produce force in greater magnitude, range and speed (Kellis, 2018). For instance, BFlh and SM have greater force generation capacity due to their greater pennation angle and physiological cross-sectional area, while ST and BFsh have greater excursion capacity, since they have longer fascicles with respect to their muscle length (Kellis, 2018). But there are not only differences between muscles, but different parts of the same muscle can also vary.
Certain changes in BFlh structure make it more vulnerable to injury during high intensity running:
Tendon anatomy. Theoretically, a tendon with greater cross-sectional area has greater stiffness, while a longer tendon has greater excursion capacity. BFlh and SM have thicker proximal free tendons (Kellis, 2018) and BFlh proximal tendon has an intermediate proximal length with respect to the other two muscles (Storey, et al., 2015). Therefore, BFlh would have a high capacity to generate force, but with a lower elongation capacity compared to SM and ST. Besides, the most proximal part of BFlh is composed of tendon (Kellis, 2018), thus, force transmission will cause more strain in this part of the muscle.
Musculo-tendinous junction (MTJ). That is how the connection between a muscle and its tendon is called. It is a point of force concentration due to differences in compliance between the muscle and tendon fibers and usually muscles tears are localized in this area. Muscles with a larger junction are more effective transmitting forces and are less prone to fail (Storey, et al., 2015). ST has the smaller MTJ, but due its composition, with a tendinous intramuscular inscription (Image 11), seems to have the capacity to dissipate forces. Unlike, BFlh, with an intermediate MTJ area, does not have the same muscular composition and consequently, could have a mechanical disadvantage for forces dissipation (Storey, et al., 2015).
Muscle fibers anatomy. Proximal BFlh fascicles are less pennated and longer than distal, with the most proximal part displaying the largest mean fascicle length (Tosovic, et al., 2016). This means that the most proximal part will have the biggest elongation capacity within the muscle, but due to a less pennated angle of the muscle fibers, it will also have less capacity to elongate before experiencing a rupture (Tosovic, et al., 2016).
Aponeuroses variation. Several articles have shown that BFlh does not have a uniform architecture and its parts vary from each other. The proximal aponeurosis has been shown to be longer and narrower than the distal one. Based on computational models, Rehorn and Blemker (2010) concluded that this fact has a great influence on the stretch distribution within the muscle, so this non-uniform muscular aponeurosis architecture will cause greater strain in the MTJ on the region with the narrowest aponeurosis.
Risk factors
In every article I´ve read about hamstring injuries risks there are two factors in which there is general agreement: Age and previous hamstring injury. Older (>25) and previously injured players have higher injury risk (Heer, et al., 2019) and unfortunately, we can´t change these factors.
Many more factors have been investigated and there is still much controversy. In a recent review with meta-analysis, 179 different hamstring strain injuries associated factors were evaluated, of which 49 demonstrated evidence of this association and 18 had conflicting results (Green, et al., 2020). Logically we can´t control all of them, so we must know how to prioritize and organize our work.
As I have already said, age and previous injuries are the strongest risk factors. However, it seems that not only previous hamstring injuries, but previous knee, ankle and calf injuries have been shown to be associated too (Green, et al., 2020).
Strength have been measured with quite ambiguous results. Although hamstring muscles strength seems to play a crucial role in injury prevention and deficits are associated with hamstring strain injuries (Green, et al., 2020), evaluation methods differ a lot between each other, as well as the results and associations with hamstring injuries. Some authors point to strength imbalances as a risk factor (Heer, et al., 2019), while others suggest that only eccentric (Breno, et al., 2020) or concentric (Shalaj, et al., 2020) strength influences injury risk.
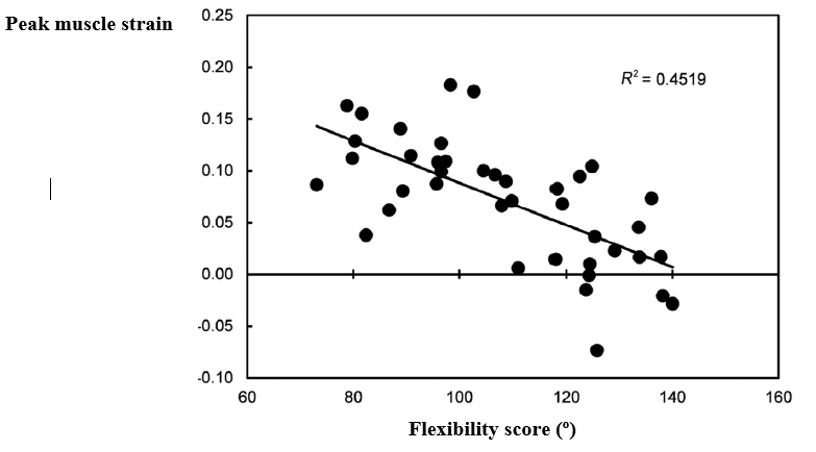
Image 12. BF peak strain during sprinting and flexibility score (Passive Straight Leg Raise). The largest peaks during sprint are related to lower range of motions in the test. (Wan, et al., 2017)
Others simply find no relationship between strength and hamstring strain injuries (Tokutake, et al., 2018). Furthermore, used assessments and measures differ too. Dynamometers (Ishoi, et al., 2019), isokinetic devices (Breno, et al., 2020) or tests as the Nordic Hamstring Strength Test (Shalaj, et al., 2020) have been used to evaluate the strength in different articles, what makes the comparison of the results quite difficult. Despite the differences, strength training will be a fundamental part in the rehabilitation process.
Flexibility and range of motion show conflicting results too. A clear relationship between flexibility, mobility, or range of motion factors with hamstring injuries has not been demonstrated (Green, et al., 2020), but although hamstring shortening is not causally related to injury, it is to increased muscle tension in shorter ranges of motion (Wan, et al., 2017). This means that a player with shortened hamstrings will experience higher strain in a shorter range of motion during high intensity actions (Image 12).
Actually, research has already shown more powerful and less flexible football players to be at greater risk of sustaining a hamstring injury (Henderson, et al., 2010), which further supports the relationship between flexibility and injury risk.
Other important factors are those related to motor control. For example, increases in hip flexor activity increase the stretch experienced by the contralateral biceps femoris during the late swing phase (Shield & Bourne, 2018). Although more research is needed in this topic, lack of neuromuscular control of lumboabdominal muscles has been associated to increased injury risk too.
A correct functioning of the gluteal muscles is essential to protect the hamstrings (Edouard, et al., 2018). For instance, weakness or wrong activation pattern of the major glute relative to hamstrings and low back muscles in tasks as the Prone Hip Extension (Image 13), are also associated with hamstring injury risk (Schuermans, et al., 2017).
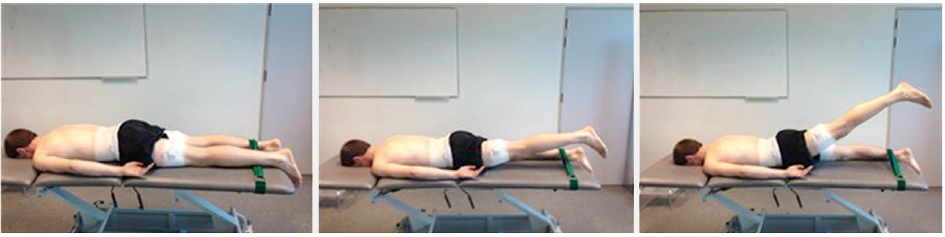
Image 13. Prone hip extension test. (Schuermans, et al., 2017)
Gluteus medium and minimum will also have a protective function, not only on the hamstrings, but also on knees, low back and lumbopelvic-hip complex (Buckthorpe, et al., 2019).
Previous injured athletes also show different kinematics during running compared to non-previously injured. Daly et al. (2016) showed an increase in anterior pelvic tilt and hip flexion during late swing and a greater knee medial rotation during early stance in athletes who had suffered hamstrings injuries in the past. Moreover, in a review from last year, Danielsson et al. showed two studies which reported that running with a forward trunk lean can increase hamstring injury risk.
Competition demands and the player´s desire to play again, can cause us to rush too much, but previous research has shown that sudden big changes in training load are related to increased injury risk (Gabbet, 2016). Wrong “Return to Play” planification could be one of the reasons for the high recurrence rate in hamstring injuries. Further, high-speed running exposure has been also associated with increased risk (Green, et al., 2020). However, players who are used to train high intensity actions show a lower injury rate, so sprint training could also provide a protective effect against injuries.
Myofascial chains
Even though everything I have written about before has focused on the behavior of the hamstrings as an isolated muscle group, this is far from the reality. Our body is not made up of isolated parts that act separately to perform the actions we need, but quite the opposite. The human body is a complex system in which all its components interact at the same time, adapting to the demands that the system, as a whole, requires or needs. Once we have restored the movement and functionality of the injured area, the athlete must adapt again to perform specific sport movements, correcting and integrating the new motor patterns that they have learned during rehabilitation.
There is a biomechanical approach related to what I just said from which we can take in many interesting ideas about injury rehabilitation and sports performance: Myofascial chains. This approach assumes that the muscles of the human body do not function as independent units but as part of a tensegrity-like, body-wide network with fascial structures acting as linking components (Wilke, et al., 2016). Up to 11 muscle chains or myofascial meridians have been identified.
The superficial back line (Image 14) connects the hamstrings with several muscles of the posterior part of the body in a long line which runs from the frontal bone in the head to the plantar fascia (Wilke, et al., 2016). But the important fact about this connection is the force transmission between components, as movement or tension in one part will affect the others (Otoni do Carmo, et al., 2013).
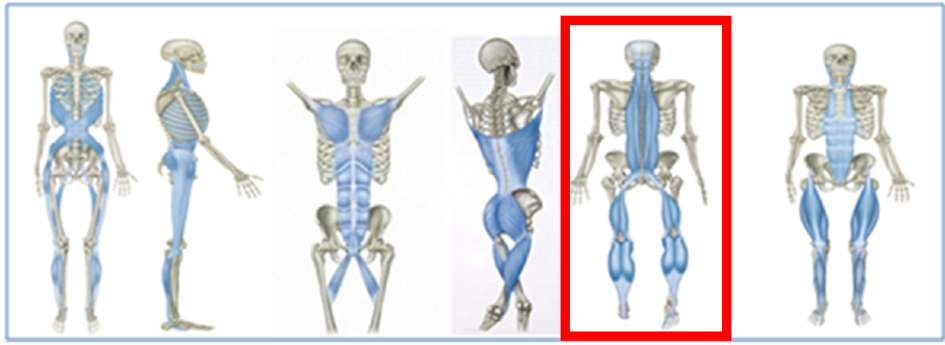
Image 14. Myofascial meridians (Superficial back line is highlighted) (Wilke, et al., 2016)
Wilke and Tenberg (2020) investigated how the movement of a muscle affects fascial tissue and its transmission. They evaluated the muscular and fascial displacement of the SM muscle during passive movement of the ankle. They found a strong correlation between fascial movement and muscle displacement and also confirmed the force transmission between the gastrocnemius and the hamstrings.
Ankle mobility is an important factor in lower limb injuries prevention and, if we think about the conclusions of the research above, mobility restrictions of this joint will increase the muscular tension of the posterior chain, something that doesn’t suit well the hamstrings.
Force transmission not only occurs in adjacent muscles, but throughout the entire muscle chain. For instance, Cruz-Montecinos et al. (2015) reported a high correlation between pelvis motion and the displacement of the deep fascia of the medial gastrocnemius (Image 15), and even the influence of hamstrings flexibility on the thoracic posture during maximum trunk flexions has been recorded (Miñarro & Alacid, 2010). Although it is sometimes necessary to train certain parts of the chain in isolation to correct deficits, our work will not be complete until we achieve efficient movement of the entire muscle chain.
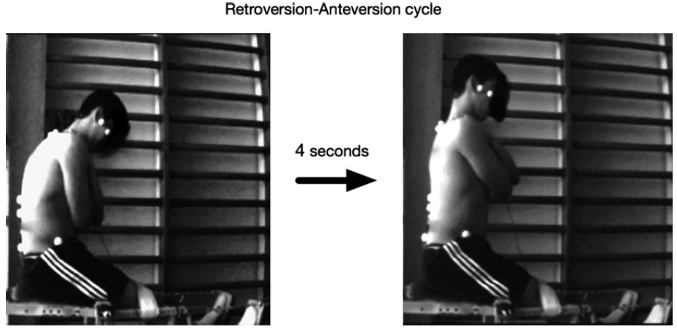
Image 15. Displacement of medial gastrocnemius fascia during pelvic motion. (Cruz-Montecinos, et al., 2015)
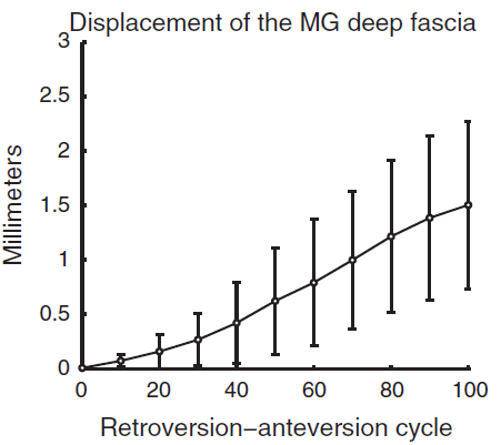
In my opinion, yes, our body has a great capacity to adapt and, therefore, it will compensate for the lack of movement or strength of one part of the body with another. The problem is that these offsets will cause incorrect movement patterns which, in many cases, will lead to an injury in the weakest part of the chain, the hamstrings.
Having enough knowledge about anatomy and biomechanics of our body will help us enormously both in injury prevention and rehabilitation. Despite the increase in the number of publications and research, hamstrings injuries continue to have a high incidence in soccer players and athletes who participate in sports based on high intensity running actions. The hamstrings are still our weak point. If we want to solve the puzzle, we must first know the pieces.
References
Adstrum, S. y otros, 2017. Defining the fascial system. Journal of bodywork and movement therapies, Volumen 21, pp. 173-177.
Askling, C. & Saartok, T., 2008. Proximal hamstring strains of stretching type in different sports: Injury situations, clinical and magnetic resonance imaging characteristics and return to sport. The american journal of sports medicine, 10(10).
Azmi, N. L., Ding, Z., Xu, R. & Bull, A., 2018. Activation of biceps femoris long head reduces tibiofemoral anterior shear force and tibial internal rotation torque in healthy subjects. Plos One, 13(1).
Benninger, B. & Delamarter, T., 2013. Distal semimembranosus muscle-tendon-unit review: morphology, accurate terminology and clinical relevance. Folia Morphologica, 72(1), pp. 1-9.
Bordoni, B. & Myers, T., 2020. A Review of the Theoretical Fascial Models: Biotensegrity, fascintegrity and myofascial chains. Cureus, 12(2).
Breno, J. y otros, 2020. Prevalence of hamstring strain injury risk factors in professional and under-20 male football (soccer) players. Journal of Sport Rehabilitation, Volumen 29, pp. 339-345.
Buckthorpe, M., Stride, M. & Della Villa, F., 2019. Assessing and treating gluteus maximus weakness – A clinical commentary. The International Journal of Sports Physical Therapy, pp. 655-670.
Chen, H.-N., Yang, K., Dong, Q.-R. & Wang, Y., 2014. Assessment of tibial rotation and meniscal movement using kinematic magnetic resonance imaging. Journal of Orthopaedic Surgery and Research, 9(65).
Cohen, S., 2015. Epidemiology, diagnosis and treatment of neck pain. Symposium on pain medicine, 90(2), pp. 284-299.
Cruz-Montecinos, y otros, 2015. In vivo relationship between pelvis motion and deep fascia displacement of the medial gastrocnemius: Anatomical and functional implications. Journal of Anatomy, Volumen 227, pp. 665-672.
Daly, C. y otros, 2016. The biomechanics of running in athletes with previous hamstring injury: A case-control study. Scandinavian Journal of Medicine and Science in Sports, Volumen 26, pp. 413-420.
Danielsson, A. y otros, 2020. The mechanism of hamstring injuries: A systematic review. BMC Musculoskeletal disorders, 21(641).
De Maeseneer, M. y otros, 2014. Distal insertions of the semi membranosus tendon: MRI imaging with anatomic correlation. skeletal radiology, Issue 43, pp. 781-791.
Dischiavi, S., Wright, A., Hegedus, E. & Bleakley, C., 2018. Biotensegrity and myofascial chains: A global approach to an integrated kinetic chain. Medical Hypotheses, pp. 90-96.
Edouard, P. y otros, 2018. Sprint acceleration mechanics in fatigue conditions: Compensatory role of gluteal muscles in horizontal force production and potential protection of hamstring muscles. Frontiers in Physiology.
Ekstrand, J., Bengtsson, H., Waldén, M. & Davison, M., 2022. Hamstring injury rates have increased during recent seasons and now constitute 24% of all injuries in men’s professional football: the UEFA Elite Club Injury Study from 2001/02 to 2021/22. British Journal of Sports Medicine, Volumen 0, pp. 1-7.
Ekstrand, J., Waldén, M. & Hägglund, M., 2016. Hamstring injuries have increased by 4% annually in men’s professional football, since 2001: a 13-year longitudinal analysis of the UEFA Elite Club injury study. British Journal of Sport Medicine, p. 731–737.
Gabbet, T., 2016. The training-injury prevention paradox: Should athletes be training smarter and harder?. The British Journal of Sports Medicine, Volumen 50, pp. 273-280.
Green, B., Bourne, M., Van Dyk, N. & Pizzari, T., 2020. Recalibrating the risk of hamstring strain injury (HSI): A 2020 systematic review and meta-analysis of risk factors for index and recurrent hamstring strain injury in sport. British Journal of Sport Medicine, Volumen 54, pp. 1081-1088.
Heer, S. y otros, 2019. Hamstring injuries. Risk factors, treatment and rehabilitation. The Journal of Bone and Joint Surgery, Volumen 101, pp. 843-853.
Henderson, G., Barnes, C. & Portas, M., 2010. Factors associated with increased propensity for hamstring injury in English Premier League soccer player. Journal of Science and Medicine in Sport, Volumen 13, pp. 397-402.
Huygaerts, S. y otros, 2020. Mechanism of hamstring strain injury: Interactions between fatigue, muscle activation and function. Sports, p. 65.
Ishoi, L. y otros, 2019. The influence of hamstring muscle peak torque and rate of torque development for sprinting performance in football players: A cross-sectional study. International journal of sports physiology and performance, Volumen 14, pp. 665-673.
James, E., LaPrade, C. & Robert, L., 2015. Anatomy and biomechanics of the lateral side of the knee and surgical implications. Sports medicine and arthroscopy review, 23(1), pp. 2-9.
Kellis, E., 2018. Intra- and inter-muscular variations in hamstring architecture and mechanics and their implications for injury: A narrative review. Sports Medicine, Volumen 48, pp. 2271-2283.
Kenneally-Dabrowski, C. y otros, 2019. Late swing or early stance? A narrative review of hamstring injury mechanisms during high-speed running. Scandinavian journal of medicine sciences and sports, Volumen 29, pp. 1083-1091.
LaPrade, M., Kennedy, M., Wijdicks, C. & LaPrade, R., 2015. Anatomy and biomechanics of the medial side of the knee and their surgical implications. Sports medicine and arthroscopy review, 23(2), pp. 63-70.
Liu, Y., Sun, Y., Zhu, W. & Yu, J., 2017. The late swing and early stance of sprinting are most hazardous for hamstring injuries. Journal of Sport and Health Science, Issue 6, pp. 133-136.
Miñarro, P. & Alacid, F., 2010. Influence of hamstring muscle extensibility on spinal curvatures in young athletes. Science and Sport, Volumen 25, pp. 188-193.
Morin, J. B. y otros, 2015. Sprint acceleration mechanics: The major role of hamstrings in horizontal force production. Frontiers in physiology, 6(404).
Orchard, J., 2012. Hamstrings are more susceptible to injury during the early stance phase of sprinting. British journal of sport medicine, 46(2).
Otoni do Carmo, V. y otros, 2013. Myofascial force transmission between the latissimus dorsi and gluteus maximus muscles: An in vivo experiment. Journal of biomechanics, pp. 1003-1007.
Panayi, S., 2010. The need for lumbar-pelvic assessment in the resolution of chronic hasmtring pain. Journal of bodywork and movement therapies, Volumen 14, pp. 294-298.
Rehorn, M. & Blemker, S., 2010. The effects of aponeurosis geometry on strain injury susceptibility explored with a 3D muscle model.. Journal of biomechanics, 43(13), p. 2574–2581.
Rodgers, C. & Raja, A., 2020. Anatomy, Bony Pelvis and Lower Limb, Hamstring Muscle. StatPearls.
Rouissi, M. y otros, 2016. Effect of leg dominance on change of direction ability amongst young elite soccer players. Jounal of sports sciences, 34(6), pp. 542-548.
Schache, A. y otros, 2012. Mechanics of the Human Hamstring Muscles during Sprinting. Medicine and science in sports and exercise, 44(4), p. 647–658.
Schuermans, J., Van Tiggelen, D. & Witvrouw, E., 2017. Prone Hip Extension Muscle Recruitment is Associated with Hamstring Injury Risk in Amateur Soccer. International Journal of Sports Medicine, pp. 696- 706.
Shalaj, I. y otros, 2020. Potential prognostic factors for hamstring muscle injury in elite male soccer players: A prospective study. Plos One, 15(11).
Shield, A. & Bourne, M., 2018. Hamstring Injury Prevention Practices in Elite Sport: Evidence for Eccentric Strength vs. Lumbo-Pelvic Training. Sports Medicine, pp. 513-524.
Stepien, K. y otros, 2019. Anatomy of proximal attachtment, course, and innervation of hamstring muscles: a pictorial essay. Knee Surgery, Sports Traumatology, Arthroscopy, Issue 27, pp. 673-684.
Storey, R., Meikle, G., Stringer, M. & Woodley, S., 2015. Proximal hamstring morphology and morphometry in men: an anatomic and MRI investigation. Scandinavian Journal of Medicine and Science in Sports, 26(12), p. 1480–1489.
Tokutake, G. y otros, 2018. The Risk Factors of Hamstring Strain Injury Induced by High-Speed Running. Journal of Sports Science and Medicine, Volumen 17, pp. 650-655.
Toor, A. y otros, 2019. The significant effect of the medial hamstrings on dynamic knee stability. Knee Surgery, Sports Traumatology, Arthroscopy, Volumen 27, p. 2608–2616.
Tosovic, D., Muirhead, J., Brown, J. & Woodley, S., 2016. Anatomy of the long head of biceps femoris: An ultrasound study. Clinical anatomy, 29(6).
Van Hooren, B. & Bosch, F., 2016. Influence of muscle slack on high intensity sport performance: A review. Strength and conditioning journal, 38(5), pp. 75-87.
Van Hooren, B. & Bosch, F., 2017. Is there really an eccentric action of the hasmtrings during the swing phase of high-speed running? Part I. A critical review of the literature. Journal of Sport Science, 35(23), pp. 2313-2321.
Wan, X. y otros, 2017. The effect of hamstring flexibility on peak hamstring muscle strain in sprinting. Journal of Sport and Health Science, Volumen 6, pp. 283-289.
Wilke, J., Krause, F., Vogt, L. & Banzer, W., 2016. What is evidence-based about myofascial chains: A systematic review. Archives of physical medicine and rehabilitation, pp. 454-461.
Wilke, J. & Tenberg, S., 2020. Semimembranosus muscle displacement is associated with movement of the superficial fascia: An in vivo ultrasound investigation. Journal of Anatomy, Volumen 237, pp. 1026-1031.
Very nice piece Raúl, interesting to hear and see your vision on hamstring injuries, founded by some good evidence. Looking forward to hearing more and discussing some of the elements 😉 .